New brain imaging technologies
New MRI Technique Tracks Brain Activity at Millisecond Timescales
A new approach to magnetic resonance imaging could allow neuroscientists to noninvasively track the propagation of brain signals on millisecond timescales, according to a study published yesterday (October 13) in Science.
The technique, which its creators call “direct imaging of neuronal activity” (DIANA), uses existing magnetic resonance imaging (MRI) technology to take series of quickfire, partial images, and then combines those images to create a high-resolution picture of which bits of the brain are active when.
DIANA has so far only been tested in anesthetized mice, and the mechanisms underlying it aren’t entirely clear, notes Matthew Self, a neuroscientist at the Netherlands Institute for Neuroscience who wasn’t involved in the work. But provided it can be replicated in other labs, the method could represent a “major advance” in brain imaging, he says.
“This would be the first technique which would be able to noninvasively measure neural activity with both a high spatial and temporal resolution,” explains Self. “I’m definitely very keen to try it.”
Researchers who spoke to The Scientist say they are already enthusiastic about the new technique’s potential.
MRI technology uses magnetic fields and radio waves to produce detailed images of tissue. Its use relies on the fact that different materials have distinct magnetic properties, allowing a scanner to distinguish between different tissues or to monitor changes in tissue over time.
Researchers have long used one version of this technology, known as blood oxygen level–dependent functional MRI (BOLD fMRI), to study how the human brain works. This method detects changes in blood flow to particular regions of the brain as a proxy for neuronal activity.
BOLD fMRI can pinpoint activity to a millimeter or less of brain tissue. But the technique’s temporal resolution is less impressive. Changes in blood flow occur over seconds—far slower than the millisecond timescale of neuronal signals. Images from fMRI will often show a whole neural pathway active all at once, when really, there’s a neural signal propagating from one part of the pathway to the next.
See “Which Neurons Go to Sleep First in Humans? fMRI Can Tell”
Other noninvasive techniques that directly measure electrical activity, such as electroencephalography (EEG) and magnetoencephalography (MEG), are much better at pinpointing the timing of neuronal firing, but much worse when it comes to spatial resolution.
In the new study, Jang-Yeon Park, a biomedical engineer at Sungkyunkwan University in South Korea, and his colleagues came up with a novel way to tackle the problem. Rather than taking full images of a particular cross-section of the brain every few seconds, as in conventional fMRI, he and his colleagues set their MRI equipment so that it would gather sequences of much smaller, partial images at very short intervals—just a few milliseconds apart. They’d then be able to stitch together these partial images to get a full view of that brain cross-section at each timepoint.
To see if they could identify any signal of brain activity with this approach, the researchers popped anesthetized mice into the MRI scanner, then lightly zapped the animals’ whisker pads with an electric current. They found that the images produced by their technique were registering some sort of signal in the somatosensory cortex—the bit of the mouse brain that senses whisker stimulation—in the 25 milliseconds or so after the zap.
Exploring this further, they discovered that the “DIANA signal” actually moved around over time. It appeared in a brain region called the thalamus about 10 milliseconds after the whisker zap, moved to one section of the somatosensory cortex at around the 25 millisecond second mark, and then sprung up in another part of the somatosensory cortex a few milliseconds later.
A bigger puzzle is what DIANA is detecting, exactly.
By taking measurements of the same brain area with invasive techniques such as electrophysiology and optogenetics, the team showed that their DIANA signal was in fact tracing the propagation of neuronal activity in response to whisker stimulation.
Peter Bandettini, a neuroscientist and physicist at the National Institute of Mental Health (NIMH) who was not involved in the study, calls the team’s work “incredibly convincing. ” Several teams have attempted to boost MRI’s temporal resolution before, he adds, but few have gone to such lengths to bolster their claims. The paper included a “tour de force of experimentation” to show that the technique was indeed tracking the propagation of neuronal signals.
Park tells The Scientist that he isn’t sure why researchers haven’t reported this effect before, given that it doesn’t require particularly special equipment, but says it’s likely that people just didn’t think to create images in this way. Bandettini notes that hacking an MRI machine to take rapid partial images like DIANA requires a significant amount of expertise—and a belief that it might turn up something interesting.
A bigger puzzle is what DIANA is detecting, exactly. Park and colleagues show in their study that BOLD effects are unlikely to be responsible, and suggest instead that their method is registering changes in the membrane potential of firing neurons, perhaps via fluctuations in the amount of water on the membrane’s surface or via cell swelling.
That’s a possibility, says Self, but overall, “the mechanism isn’t super clear. . . . I think that needs to be demonstrated in future studies.”
In its current form, DIANA has a few limitations, notes Park, who is named as co-inventor on a patent related to the method. Due to the way it stiches together full-brain snapshots by combining partial images taken at different times, the technique is likely to be susceptible to so-called motion artifacts—disruptions caused by the animal moving its head between takes. That could present some challenges in translating DIANA to awake animals or people.
The signal that DIANA is picking up is also relatively weak—around an order of magnitude smaller than that in BOLD fMRI, notes Bandettini. Groups would need relatively sophisticated MRI equipment to be able to mimic the team’s approach, as well as an experimental protocol that included repeat tasks or stimulations to allow for averaging results from multiple scans, he says. “You need a lot of repetitions of the same thing, and very, very precise resolution. ”
However, researchers who spoke to The Scientist say they are already enthusiastic about the new technique’s potential. Bandettini points to another of the team’s experiments that suggests DIANA may be able to distinguish between excitatory and inhibitory neuronal signals—something that is challenging even with invasive techniques such as electrophysiology. “That’s super exciting. That would open up an entire area of understanding how the brain interacts.”
Self, who studies visual processing, says that he knows of several groups already trying to put DIANA to work in people. Although the technique still can’t offer the single-cell resolution achievable with some invasive technologies, there are wide-reaching implications if it works in other labs, he says. “In principle, it could be taken into humans, it could be taken perhaps even into patient studies—it could open up a whole world of research into understanding the brain in health and disease.”
Breakthrough in brain imaging may offer future alternative to functional MRI
Keck School of Medicine of USC researchers, working with a Caltech team, demonstrate possibilities of innovative imaging technology to visualize brain function.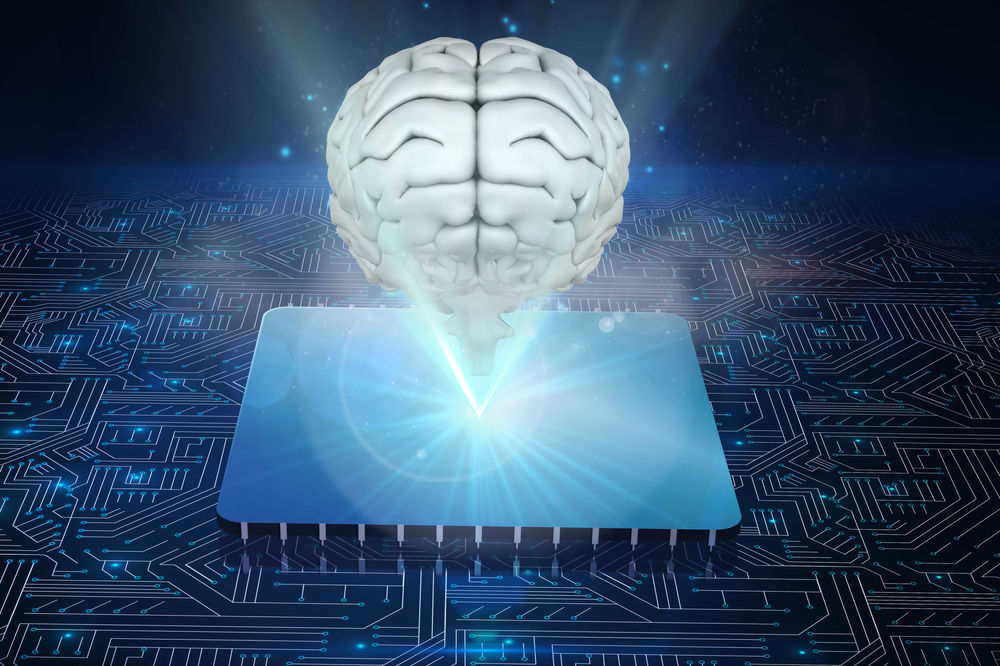
By Jeremy Deutchman
Charles Liu and Jonathan Russin
The gold standard in functional brain imaging for over two decades, functional magnetic resonance imaging (fMRI) has transformed the landscape of research and clinical care. Yet, because of its cost and functional limitations, scientists have continued to look for new ways to see into the human brain.
Researchers from the Keck School of Medicine of USC and the California Institute of Technology (Caltech), with the help of patients recovering from traumatic brain injury, have now demonstrated an alternative way to produce highly detailed images of the human brain. Their work, published in Nature Biomedical Engineering, yielded the first pictures of human brain function ever produced using functional photoacoustic computerized tomography (fPACT) – a landmark in the history of functional brain imaging.
Technology meets technique
Imaging with fPACT works by emitting a beam of laser light into the area being imaged. The light is absorbed by oxygen-carrying hemoglobin molecules in the patient’s red blood cells causing them to vibrate ultrasonically. The vibrations can then be scanned by sensors. Lihong Wang, PhD the Bren professor of medical and electrical engineering at the Andrew and Peggy Cherng Department of Medical Engineering at Caltech is a leader in the field of photoacoustic imaging.
The technology has previously been successful in animal and some human tissue models, but there are hurdles to applying it to the brain. “The human skull is an acoustic lens, but it distorts and attenuates our signals,” Wang says. “It’s like looking outside through a wavy window. The barrier impacts the clarity of the image rendered, a challenge our field is working to overcome.”
Despite the challenges, this research collaboration has shown proof of concept that fPACT can be used for brain imaging. To establish the technology’s effectiveness, Charles Liu, MD, PhD, director of the USC Neurorestoration Center and professor of clinical neurological surgery, and Jonathan Russin, MD, an associate director of the USC Neurorestoration Center and assistant professor of clinical neurological surgery, enlisted the aid of traumatic brain injury patients in the USC Neurorestoration Center.
“Our study subjects had undergone hemicraniectomy [the temporary removal of a large portion of the skull], which enabled us to test the technology without interference in fully awake humans prior to skull reconstruction surgery. Thanks to their participation, we discovered fPACT is capable of creating functional brain images that are superior in some ways to 7T fMRI,” Liu says. “This may be something that will change neuroimaging forever.”
The study subjects were recruited from Rancho Los Amigos National Rehabilitation Center where a large number of cranial reconstruction surgeries are performed. “It is terrific to work with patients to develop transformative tools to better understand how to treat their neurological disabilities”, adds Liu, who also serves as chair of neurosurgery and orthopedics and chief of innovation and research at Rancho.
The benefits could be enormous. “fPACT costs less than MRI, is potentially portable, is accessible to patients with implants and eliminates the claustrophobic/magnetic MRI environment,” Russin notes.
“This study is a first for functional human brain imaging – and, we believe, a critical step forward in advancing the field.”
Reactions to results
In order to complete the study, Liu, Russin and Wang solicited critical input from the USC Mark and Mary Stevens Institute for Neuroimaging and Informatics, which provided expertise and resources including access to a state-of-the-art 7-Tesla MRI. “To understand the potential of fPACT, the research team needed to compare it to the best current functional brain imaging,” says Danny JJ Wang, PhD, professor of neurology and radiology. “After reviewing the data, it seems clear that fPACT could have immense impact in neuroscience, with applications ranging from vessel and tumor imaging to localization of function and seizures.”
Results from the study highlight fPACT’s ability to produce accurate 3D maps of blood flow. Since blood flow increases to specific areas of the brain during cognitive tasks, a device that shows blood concentration and oxygenation changes can help researchers and medical professionals monitor brain activity. This is known as functional imaging.
The study offers key insight into fPACT’s long-term potential. Today, Liu, Russin and their team are partnering with Caltech to develop workarounds to skull interference to further investigate the possibilities of fPACT technology.
About the study
This study was a collaborative effort among expert researchers across multiple disciplines. Additional co-authors include Kay Jann, and Lirong Yan from the Keck School of Medicine of USC; Shuai Na, Li Lin, Xiaoyun Yuan (currently at Tsinghua University), Peng Hu, Junhui Shi (currently at Zhijiang Laboratories), and Konstantin Maslov from the California Institute of Technology.
New 3D visualization shows how the human brain pulsates
Science May 6, 2021
Further
Anastasia Nikiforova news editor nine0003
Anastasia Nikiforova news editor
A new imaging technique captures brain movements with stunning detail and has diagnostic potential.
Read Hi-Tech in
Stevens Institute of Technology scientists have developed a new imaging method. It allows you to get an incredibly detailed three-dimensional video of the human brain. The method offers clinicians a unique diagnostic tool and researchers new insights into neurological disorders. nine0003
Back in 2016, an innovative imaging technology, enhanced magnetic resonance imaging (aMRI), appeared on the market. The method allowed researchers and clinicians to observe the pulsating movements of the brain in real time, giving insight into the biochemical reactions of the organ tissue.
Scientists have now improved 2D visualization. The 3D format provides doctors with a detailed picture of brain movement in three dimensions.
New 3D aMRI technology provides unsurpassed spatial resolution, the authors note. It allows you to visualize movement in the brain with an unprecedented level of detail. The scientists also note that the technology will allow creating completely new types of brain movement models. The new understanding of how fluid moves in the body will help further research into various brain diseases.
Read more
The first accurate map of the world has been created. What's wrong with everyone else? nine0003
Elon Musk: the first tourists to Mars will die
Read more
New Imaging Technology Offers Incredibly Detailed 3D Videos of a Pulsating Brain
A new imaging technique has been developed to provide incredibly detailed 3D video of the human brain. This method offers unprecedented perspectives on pulsatile brain movements, providing clinicians with a new diagnostic tool and researchers with a new perspective on neurological disorders. nine0003
Back in 2016, an innovative imaging technology called enhanced magnetic resonance imaging (aMRI) was introduced. This method allowed researchers and clinicians to observe the pulsating movements of the brain in real time, giving insight into the biochemical reactions of brain tissue.
Initially offering only 2D visualization, the researchers have now improved the technique to offer impressive 3D animation. This gives doctors a detailed picture of brain movement in three dimensions for the first time. nine0003
"The new method magnifies the microscopic rhythmic pulsations of the brain during the heartbeat, allowing the visualization of tiny piston movements that are smaller than the width of a human hair," explains Itamer Terem, a Stanford University student working on the project. . "The new 3D version provides a larger magnification factor, which gives us better visibility into brain movements and better accuracy."
3D aMRI CSF and Whole Brain
New 3D aMRI technology delivers unparalleled spatial resolution, allowing movement in the brain to be visualized at an unprecedented level of detail, promising benefits for both researchers and clinicians. nine0003
“We are using 3D aMRI to see if we can find new insights into the impact of mild traumatic brain injury on the brain,” explains Samantha Holdsworth, a New Zealand researcher who worked on the original 2D aMRI technology. “One study is already underway, a collaboration between Matai and the University of Auckland using 3D magnetic resonance imaging along with brain modeling techniques to see if we can develop a non-invasive way to measure pressure in the brain, which in some cases could eliminate the need for brain surgery.