How does chronic stress change your dna
How chronic stress is harming our DNA
Money problems, a heavy work load, caregiving — such increasingly common pressures have helped make stress a part of modern American life. According to APA's Stress in America survey, 42 percent of adults in the United States. say their stress level has increased over the past five years. Even teens reported stress rivaling adult levels.
Recent research suggests chronic stress damage starts before we're even conceived and cuts into our very cells. A number of studies have linked stress with shorter telomeres, a chromosome component that's been associated with cellular aging and risk for heart disease, diabetes and cancer.
How do personality and environment play into this phenomenon? Elissa Epel, PhD, has been exploring that question for more than a decade at the University of California, San Francisco, where she directs the Center for Aging, Metabolism and Emotion. She often works with Elizabeth Blackburn, PhD, who won a Nobel Prize in 2009 for her research on telomeres.
Epel talked to the Monitor about her quest to understand stress and what might help lessen its effects.
What are telomeres and how are they related to aging and disease?
Telomeres are a protective casing at the end of a strand of DNA. Each time a cell divides, it loses a bit of its telomeres. An enzyme called telomerase can replenish it, but chronic stress and cortisol exposure decrease your supply. When the telomere is too diminished, the cell often dies or becomes pro-inflammatory. This sets the aging process in motion, along with associated health risks.
How does stress rank in terms of factors that affect telomere length?
The two biggest factors are chronological aging and genetics, but stress is now on the map as one of the most consistent predictors of shorter telomere length. The type of stress determines how big its effect is. It seems exposures to multiple early life adversities, such as child neglect, have the largest effects, since they track through to late adulthood, or they set in place persistent mechanisms that maintain short telomeres throughout life, such as exaggerated stress reactivity and poor health behaviors. Stressors such as caregiving in late life also have an effect. So we can see this relationship between stress and cell aging across a lifespan, and it's fundamental to how we're built. Our brains are constantly looking for threats to our survival. When we expose our bodies to years of chronic stress arousal, we see effects that override normal aging, making our telomeres look like they are from a significantly older person. When we look at groups of people with psychiatric disorders related to dysregulated emotional responses, especially depression, and compare them to controls who have never experienced these disorders, they consistently have shorter telomeres.
How early in life do the negative effects of stress begin?
If you want to be literal about it, it starts before conception. A baby's intrauterine environment is shaped by a mom's pre-existing physical health. There have also been several studies looking at maternal health and telomeres in offspring. So far, we found in a small study that the higher a mom's prenatal anxiety, the shorter the baby's telomere length, as seen in the work of Sonja Entringer, PhD, Pathik Wadhwa, PhD, and others.
This scenario is setting the stage for an accelerated trajectory of aging. It may, in fact, be one of the most critical periods in time for impacting cellular aging. Transgenerational transmission of risks has to be taken into account for understanding and improving public health.
Another consistent pattern turning up in both clinical and epidemiological samples is that early life adversity is associated with shorter telomeres. This relationship was first observed in adults when early adversity was assessed retrospectively, but now it has been observed in young children prospectively. Maltreatment, abuse, severe neglect and exposure to violence all seem to take a swath from the telomeres.
The good news is that there are buffers to early adversity, such as warm and interactive high-quality parenting, or possibly the luck of having a more stress-resilient genotype, according to a small study by Colter Mitchell, PhD, and colleagues.
One of your recent studies has the intriguing finding that stress may alter how we metabolize high-fat, sugary foods.
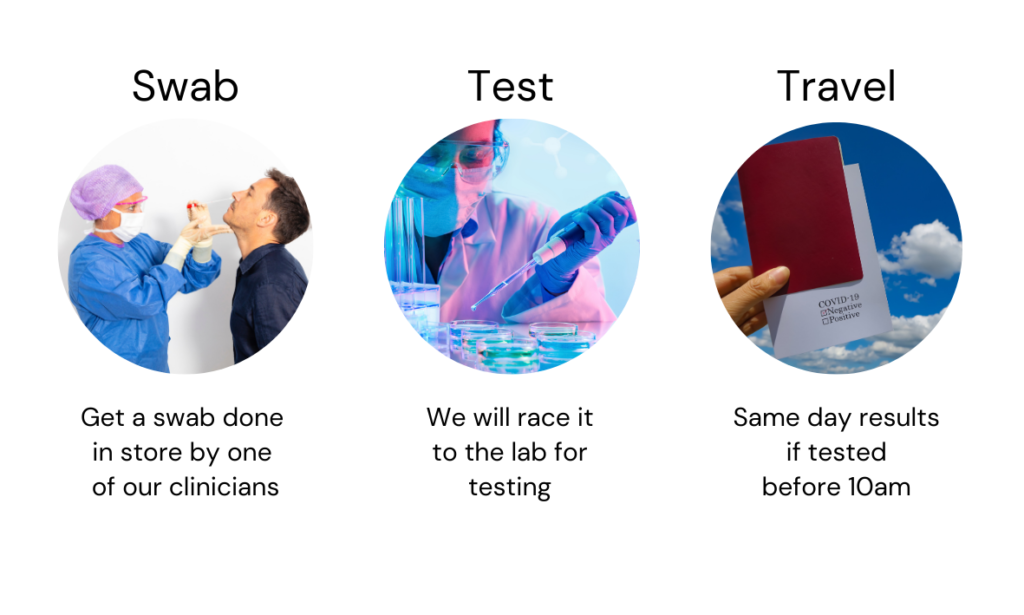
Ah, yes. There is a somewhat involved story here. Chronic stress wreaks havoc in neurally driven compulsive eating patterns. It can cause neuroplastic changes that alter how we perceive and react to the world in ways that might be good for short-term survival but not for longevity mechanisms. Stress impairs our executive function, which dampens our ability to resist impulses. Chronic stress can increase the reward responsiveness of our brains. So if we are at all prone to addiction, it's going to make us crave palatable food or drugs even more. It drives us to choose comfort foods, whether unconsciously or with a strong intention. Now what's happening in the body? We know that people with high stress develop greater levels of abdominal fat. Mouse models have shown that pathway. The combination of high stress and [eating a lot of] junk food works synergistically here. Stress leads to neuropeptide Y (NPY), a chemical that triggers the intra-abdominal fat cells to mature and fill up with more fat.
Assistant professor Kirstin Aschbacher, PhD, and I examined this in humans and found the suspected pattern. The high-stress caregivers who ate more comfort food had higher NPY and abdominal fat. But this was not observed in the caregivers with the healthier diet, nor in the low-stress controls with the junk food diet. In our next study we are putting high- versus low-stress people on a high sugar diet, short term of course, to test these effects more experimentally.
After the rodent study that looked at NPY, there was a flurry of excitement: "Can we block NPY and the stress-fat relationship?" I think this is unlikely. There are multiple stress pathways, so we'd have to block stress at its source, which is how we perceive events. Otherwise, you're putting a little Band-Aid on the robust positive feedback loop between stress biology and fat cells. That's a major survival mechanism, and I doubt it can be blocked by just one chemical pathway.
What else are you working on?
Our group, including my co-principal investigators Nancy Adler, PhD, and Barbara Laraia, PhD, has taken on the study that excites us the most: an intervention that helps overweight, low-income, pregnant women turn their health around, for themselves and their babies. We want to take advantage of pregnancy as an important "teachable moment" when motivation is high and when the mental and physical health of the next generation is being formed. But this has turned out to be the most challenging intervention we've run as well.
We've developed a mindful stress reduction/mindful eating class to reduce stress and excess weight gain during pregnancy. Despite these women's high motivation, there are structural factors that prevent them from meeting their goals. There are too many unpredictable stressful and even traumatic events embedded in their neighborhoods and social environments that continue when they're pregnant. They witness or experience violence. One of our participants was even shot in a limb while pregnant. The developing babies are exposed to all of this.
A class alone also can't change critical aspects of their food environment, including food insecurity — the stressful state of not having enough money to feed yourself and family. Our class can help them reduce their levels of depression and stress, which has meaningful biochemical sequelae, but helping them prevent excess weight gain may take more of a systemic village effort and must start earlier than mid-pregnancy to influence the health of the next generation.
I am also very focused on improving stress reduction interventions through adaptive emotion regulation — partly through mindfulness-based techniques. I have studies at residential retreat centers, as well as a study trying to teach parents mindfulness skills. While general mindfulness training is helpful and can be transformative to some, there are many ways to use it to tailor treatments, to use technology to bring awareness to one's busy day.
We are also testing the effects of aerobic exercise on telomerase in sedentary young adults — the work of assistant professor Eli Puterman, PhD, of UCSF. Exercise is probably the biggest antidote to biological stress dysregulation — excessive cortisol, insulin, inflammation and oxidative stress that make up a "stress soup." Eli has shown cross-sectionally that exercise moderates the stress-telomere relationship, and he recently published the first demonstration of what appears to be longitudinal lifestyle stress buffering. If you have a really stressful year, yes, your telomeres may take a hit, but not if you are doing the daily work of health maintenance — exercising, eating fruits and vegetables, and getting enough sleep. If you've got those habits, your telomere attrition looks like someone who glided through the year with no big stressful events.
Which risk factors for chronic stress do you think are most underappreciated?
Our social environment. How rich is your social fabric? How connected are you to the people in your social circle, starting with your family? A strong social network is probably the biggest buffer from toxic stress, next to exercise. Yet we often lack quality long-term social connections. There is frequent loneliness among high-risk groups like the elderly, who may be more isolated. For those of low income, many are working long and inflexible hours. Part of the problem is that there are limited minutes in the day, and if you are overworking, you are undercaring for your social network and for yourself.
You're starting a non-profit within UCSF dispensing tests that measure telomere length. Won't it be more stressful to find out you have short telomeres than not to know at all?
We've seen the value of telomere length as a predictor for age-related diseases. Many findings indicate it may be possible to build on that to monitor and even prevent disease rather than wait until we get news of some diagnosis we can't change. We are learning that many people want to quantify themselves and track their behaviors. Maybe knowing your telomere length and how it is changing each year could be helpful. Maybe it's worse to know. We just don't know yet.
We plan to host a large group experiment whereby people measure their telomeres at low cost over time, and provide data on the behaviors, medications, events and so on that may be affecting rate of change over time. By crowdsourcing data in this way, we can learn more about the dynamics of change in real life. Will such knowledge be empowering and motivating, and will people's efforts to lengthen telomeres even help? So far, one of our small controlled studies suggested it's not too stressful to learn you are on the short end. People want to know their telomere length, with responsible interpretation of their risks.
So we're interested in answering that question more thoroughly: How useful is this measure to individuals?
You're a mother with a demanding job managing diverse projects in multiple areas. How does that affect your own stress levels, and how do you cope?
Work stress is much more of the positive "challenge" stress than "threat" stress. It's gratifying to mentor, help people find their research identities, and get to work with wonderful collaborators and be part of the discovery process across a range of topics. But inevitably, there is too much to do with too little time. No researcher I know of escapes that. If you stay in this business, you can learn to manage that, somewhat with strategy and somewhat with mindset, so it doesn't eat away at you.
The true stress for me comes from parenting a child with special issues. Because of this, I have a good sense of the inside experience of the young and elderly caregivers I study. Compassion for their situations fuels my drive to improve interventions for caregiving stress, such as by developing tailored mindfulness classes.
One thing to remember about chronic stress is that it's only our thoughts that make it seem so. Viewed mindfully, no situation is truly chronic — there are always calm moments to notice and be present for. Moments that can be lived in with ease.
Stacy Lu is a journalist in Falls Church, Va.
Stress Hormone Causes Epigenetic Changes
You are here
Home » News & Events » NIH Research Matters
September 27, 2010
Researchers found that chronic exposure to a stress hormone causes modifications to DNA in the brains of mice, prompting changes in gene expression. The new finding provides clues into how chronic stress might affect human behavior.
During stressful situations, we produce steroid hormones called glucocorticoids that affect many systems throughout the body. These effects are mediated by the hypothalamic-pituitary-adrenal (HPA) axis, a network involving the hypothalamus and pituitary gland in the brain and the adrenal glands near the kidneys.
Past studies have found that glucocorticoids alter gene expression in the brain. A group led by Drs. James B. Potash and Gary S. Wand at the Johns Hopkins University School of Medicine set out to investigate how glucocorticoids affect genes central to the HPA axis. They hypothesized that the hormones may affect the HPA axis through epigenetic modifications—changes to DNA that don’t alter sequences but influence gene expression.
The researchers added corticosterone—the major hormone that mice produce in stressful situations—to their drinking water for 4 weeks. After exposure, and again after a 4-week recovery period without corticosterone, the scientists tested the mice for behavioral and physiological changes. They examined the expression levels of 5 HPA axis genes in the hippocampus, hypothalamus and blood. They also tested the genes' methylation levels—a common epigenetic modification that affects gene expression. The study was funded by grants from NIH's National Institute on Alcohol Abuse and Alcoholism (NIAAA), Eunice Kennedy Shriver National Institute of Child Health and Human Development (NICHD) and National Institute of Mental Health (NIMH).
In the September 2010 issue of Endocrinology, the researchers reported that mice given corticosterone appeared more anxious during a maze test. Chronic exposure to corticosterone altered the expression of 3 HPA axis genes, including higher levels of Fkbp5 in the hippocampus, hypothalamus and blood. The protein that Fkbp5 codes for is part of a molecular complex that interacts with the glucocorticoid receptor. In addition, the researchers found decreased Fkbp5 methylation levels in the mice exposed to corticosterone.
Genetic variations in Fkbp5 have been associated with posttraumatic stress disorder and mood disorders, which are characterized by abnormal glucocorticoid regulation. These results suggest that methylation of Fkbp5 may play a role in mediating the effects of glucocorticoids on behavior.
"This gets at the mechanism through which we think epigenetics is important," says Potash. Epigenetic marks added to DNA through life experience may prepare an animal for future events, he explains. "If you think of the stress system as preparing you for fight or flight, you might imagine that these epigenetic changes might prepare you to fight harder or flee faster the next time you encounter something stressful."
With modern stressors, such as work deadlines, we can’t fight or flee, and chronic stress may instead lead to depression or other mood disorders. Understanding the mechanism by which chronic stress leads to these conditions might help us find new ways to prevent or treat them in the future. This research suggests that epigenetic changes could play a role in the process. However, it’s important to note that the connection is still speculative. Future studies will be needed to better understand the effects of chronic stress.
—by Harrison Wein, Ph.D.
Related Links
- Feeling Stressed? Stress Relief Might Help Your Health
- 5 Things You Should Know About Stress
Connect with Us
- Contact Us
- YouTube
- Flickr
Can stress be inherited
Regulatory molecules that appear in spermatozoa due to stress affect the activity of genes in the embryo.
Recently, more and more often they say that the way of life of parents, their life experience affects what their descendants will be like. For example, hunger or psychological stress that the previous generation had to endure will affect the psychology and metabolism of the next generation.
Stress can be inherited - at least in mice. (Photo by Sofia Ramirez / Flickr.com.)
The main epigenetic ways that change gene activity are histone modification that changes DNA packaging, methylation of DNA itself, and recruitment of microregulatory RNAs. (Figure AJC1 / Flickr.com.)
‹
›
View full size
Two years ago, researchers at Emory University published a paper in Nature Neuroscience , in which they said that frightened mice had babies that were afraid of the same thing that their parents were afraid of. Another well-known example is the increased predisposition to diabetes and obesity in the children and grandchildren of Dutch women who survived the famous famine in the winter of 1944 years old. (Here, as we understand it, the point is not in the specific features of the Dutch famine, but in the fact that biologists and physicians paid attention to this historical situation and tried to investigate it.) Such observations have now accumulated, we repeat, there are already enough, but there is one problem here : neither hunger, nor psychological stress, nor other similar influences from the outside create mutations, do not change the genetic code expressed in the sequence of nucleotides in DNA. The question arises how then all this is inherited.
It is believed that such effects owe their existence to epigenetic mechanisms that control the activity of genes. There are several types of them, the main of which are the methylation of the nitrogenous bases of DNA, the modification of histones (DNA packaging proteins), and the action of regulatory RNAs. Both methyl groups, and modified histones, and regulatory RNAs can change the functioning of certain genes for a long time, almost for a lifetime, and such mechanisms are often activated under the influence of external factors. Moreover, according to some data, the nature of epigenetic changes can be inherited. However, how exactly this happens - and whether it happens - is still heatedly debated.
To move on to the next generation, modifications must be preserved in germ cells, but for the time being, all experiments indicated that in animals, when germ cells mature, all epigenetic marks are erased. But two years ago, an article was published in Science stating that in some parts of the DNA of germ cells, such marks are still preserved (in that article, we talked about DNA methylation). And last year, experts from the University of Zurich reported that some regulatory RNAs can serve as carriers of stressful experiences from parents to offspring: after mice were stressed, regulatory molecules appeared both in the hippocampus and blood serum, and in spermatozoa. And the cubs, which were obtained after fertilization with such spermatozoa, demonstrated in behavior and metabolism the same post-stress features that their parents had.
Everything indicated that at least the epigenetic settings associated with regulatory RNAs could be passed down from generation to generation. It remained only to directly confirm the causal relationship between such RNAs and the transmitted effect. This was done by Tracy Bale ( Tracy Bale ) and her colleagues from the University of Pennsylvania. To date, they have accumulated evidence that the offspring of males who were subjected to stress (whether it be constant white noise, or the smell of a predator, or regular restriction of mobility) react to such circumstances already weaker, which, in particular, is noticeable in a lower level stress hormone corticosterone. On the other hand, it was found that several types of regulatory RNAs accumulate in the spermatozoa of stressed paternal mice (more precisely, they are called microregulatory, microRNA, because of their small size compared to other classes of RNA).
In their new experiments, described in article PNAS , the researchers took microRNAs and injected them into fertilized eggs of normal mice, after which they were implanted in females and waited for mice to appear. Subsequently, they showed the same diminished response to stress as those born directly from frightened males. It was obvious that it was all about foreign miRNAs, because all the genetic material came from ordinary parents, who were not frightened by anything.
Usually microRNAs suppress the activity of genes. As expected, some genes in the eggs did not work after the introduction of regulatory RNAs. The authors of the work also tried to analyze the state of the hypothalamus, a brain gland that controls a huge number of physiological and behavioral reactions (from sleep and food to reproduction). The level of corticosterone, among other things, depends on the hypothalamus. Indeed, in mice grown from miRNA-treated fertilized eggs, some genes in it worked differently; curiously, they were related to collagen and extracellular matrix proteins. How this relates to the stress response is not entirely clear. It is possible that changes in the synthesis of connective tissue collagen and matrix proteins affect the permeability of the blood-brain barrier that stands between the blood and the brain - which, in turn, affects the sensitivity of the hypothalamus to stress signals.
In general, it remains to be seen how changes in genetic activity during the earliest stages of development lead to changes in response to stress. Microregulatory RNAs apparently act indirectly here: their level is not renewed every time after cell division, and in the brain of adult mice their number becomes quite normal. On the other hand, it would be interesting to find out through what molecular mechanisms stress can affect miRNAs in spermatozoa, and what happens in this case with spermatozoa of the next generations. Finally, the stress response is quite complex in structure, and different aspects of it may well be associated with different RNAs.
The data obtained are quite consistent with the results of the Zurich group, which we spoke about above: they also talked about the effect of stress, microRNA and male germ cells. By the way, not only stress, but also obesity can be transmitted through the male line, and it seems to be transmitted only to sons - researchers from Ohio University reported this several years ago (although those experiments were again done on mice). Note, however, that it is still quite far from any medical and clinical conclusions: we still do not know under what conditions epigenetic inheritance works, and how to separate “genetics” from “epigenetics” under normal, non-laboratory conditions.
15 things to know about metabolism to keep fit
April 13Health
You can boost your metabolism with water, spices and a five-minute workout.
Iya Zorina
Author of Lifehacker, athlete, CCM
Share
0RMR). This is the number of calories that the body spends on all the chemical processes necessary for life.
In other words, basal metabolism is the energy we expend when we are not doing anything at all - not moving or even digesting food.
And for most sedentary people, basal metabolism accounts for 65-70% of all daily energy expenditure. Only the remaining 30-35% comes from physical activity and digestion.
2. Your metabolic rate is largely determined by genetics
Studies of siblings and twins have shown that 40-45% of metabolism is determined by genetics. The same goes for the thermal effect of carbohydrates: how much energy you spend on digesting food depends on 40-50% of your DNA profile.
Even how many calories you burn during exercise depends in part on your genes. True, only when working with low power.
This explains why not all people are equally good at losing weight and gaining muscle on certain diets. Everything is individual, and you will have to try to find your ideal mode.
3. Metabolism slows down with age, but this can be prevented
The metabolic rate in adults gradually decreases. Starting from 18–19years, every year you spend less and less energy. A sharp decline in metabolism occurs after 30, then there is a slight rise in 50-59 years, followed by a gradual decline until the very end.
This is one of the reasons why over the years it becomes more difficult to maintain a normal weight and get rid of extra pounds.
But there is good news: this decline can be avoided.
One study tested the metabolic rate of women in different age groups: 21-35 years and 50-72 years. In inactive young and old people, the difference between the metabolic rate differed by about 10%. But in athletes running long distances and swimmers, such a difference was not found.
Although the study was conducted only on women, scientists believe that regular endurance sports will help to avoid age-related changes in people of both sexes. A great excuse to finally put on your running shoes or sign up for a pool session.
4. More muscle means faster metabolism
Skeletal muscle makes up 45-55% of body weight and is the largest contributor to energy expenditure, especially during physical activity.
Therefore, despite being overweight, obese people have a lower resting metabolism than normal weight people.
The more muscles you manage to build, the more energy your body will use to maintain them. This means that your metabolism will increase at rest.
5. Calculators can be wrong
Various formulas are used to calculate basal metabolic rate, but none of them gives an accurate idea of how much energy you actually burn and how many calories you need to consume to maintain or lose weight.
The Mifflin-San Geor and Harris-Benedict equations are considered the most reliable, but they also give errors.
The average deviation according to common formulas is from 314 to 445 kcal per day. In fact, it is a complete meal.
In addition, the more muscle mass a person has, the more the formulas are wrong, underestimating energy needs.
When the accuracy of the calculations was tested on young athletes, it turned out that the most reliable equations underestimate the daily calorie requirement by 284 kcal for women (23% body fat) and 110 kcal for men (15% body fat).
6. Lack of sleep increases metabolism, but this does not help to lose weight
During the night's rest, energy expenditure decreases, so the less you sleep, the more calories you burn per day.
One study found that people who sleep 5 hours burn 5% more calories per day than those who sleep 9 hours. In another experiment, they found that 24 hours without sleep increased energy expenditure by an average of 7% per day.
However, along with the activation of calorie consumption, the appetite also increases, as well as cravings for fatty and sweet foods. So if you do not control the diet, then after a bad night you will quickly block the extra energy expenditure with snacks.
7. Stress does not reduce metabolism, but may lead to weight gain
A recent study showed that psychological stress and anxiety levels do not affect resting metabolism.
However, chronic stress can still lead to weight gain. Constant anxiety changes the concentration of hunger and satiety hormones, forcing you to eat more than you need and lean on high-calorie foods.
In addition, stress reduces the body's ability to oxidize fats and expend energy after eating fatty foods.
![]()
If you were nervous, you will burn about 104 fewer calories in the next six hours than if everything was fine. Scientists have calculated that daily stress can result in an increase of 5 kg per year.
8. Strict Diets Slow Your Metabolism
When you drastically reduce your calorie intake and lose weight, your body goes into energy saving mode: your basal metabolism slows down and may stay that way even after you restore normal calorie intake. And a number of studies confirm this.
The slowdown in metabolism is partly due to the loss of muscle mass, which disappears along with fat, if nothing is done to preserve it. When you return to your usual diet, the weight quickly gains. Fortunately, this can be avoided by choosing the right diet.
9. A high amount of protein speeds up metabolism even on a diet
The body needs to spend much more energy on the absorption of dietary protein than on the processing of carbohydrates and fats.
About 20-30% of the calories you get from protein will go into protein digestion.
Due to this effect, protein increases resting energy expenditure, helping to maintain normal weight. What's more, a high protein intake helps maintain and even build muscle mass on the diet and thus avoid slowing down the metabolism.
10. Exercise Increases Resting Metabolism Even When Dieting
Physical activity not only increases energy expenditure during the day, but also affects resting metabolic rate, increasing it even in a calorie deficit.
And power loads do it best. They not only increase metabolism at rest and during sleep by 7-9%, but also help maintain and build muscle mass.
11. Exercising too hard can slow down metabolism
Both aerobic and strength training are equally good at supporting basal metabolic rate, but if the activity becomes exhausting, the opposite happens.
Observation of experienced cyclists showed that during the period of heavy loads - up to 150% of the usual intensity of training - resting energy expenditure is significantly reduced.
The same was noted in an experiment on elite rowers - high loads for four weeks reduced their energy consumption by an average of 111 kcal per day.
12. Spices can increase metabolism
Some spices activate thermogenesis during the digestion of food, causing the body to burn more calories. These include ginger, grains of paradise pepper and chili.
Add these spices to your food and drinks and you can burn an extra 40-50 calories per day. What’s more, ginger and chili peppers have anti-inflammatory and anti-cancer properties, which can have a positive impact on overall health.
13. Five minutes of exercise can speed up your metabolism for a day
This is about high-intensity interval training (HIIT) - alternating short periods of hard work with intervals of rest or recovery activity. For example, 20 seconds of sprinting and 10 seconds of jogging.
Due to the high intensity, HIIT burns more calories than the same time of quiet work, perfectly pumps endurance and accelerates metabolism at rest.
![]()
In one study of 10 people, short HIIT was found to increase basal metabolic rate for an entire day.
After a short warm-up, the participants did four minutes of interval training. The complex of exercises included squats, jumps in place, lunges and stretching according to the Tabata protocol: 20 seconds of work without stopping, 10 seconds of rest, and so on eight times. Finished with stretching.
In the six minutes of the session, the participants burned about 63 kcal, not that much. But when scientists calculated the increased energy expenditure over the next 24 hours, it turned out that the workout actually burned 360 calories.
This is just one small study, but the data sounds very encouraging. Now you know for sure that exercising for five minutes is much better than not exercising at all.
14. Coffee and tea increase metabolism
Two of the most common drinks help burn more calories due to the content of caffeine, a substance that stimulates the central nervous system.
One cup of brewed coffee (about 250 ml, 100 mg caffeine) increases energy expenditure by 92 kcal per hour for the next three hours after ingestion.
Green tea also contains caffeine, as well as catechins, substances with antioxidant properties. Together, they increase thermogenesis and help avoid a slowdown in metabolism, even on low-calorie diets.
15. Cold water can increase resting metabolism
Some studies confirm that plain water can increase resting energy expenditure.
For example, a study by Michael Boschmann on 14 healthy men and women without excess weight found that 500 ml of cool water (22°C) increased participants' metabolism by 30%.
People burned calories at an accelerated rate within 10 minutes after drinking, and after 30-40 minutes the effect peaked. Scientists have calculated that by drinking 2 liters of water a day, a person can burn an additional 95 kcal.
Three years later, Clive M. Brown did a similar experiment with eight healthy young adults of both sexes and got less than optimistic results.
In his study, about 500 ml of cold water (3°C) increased subjects' metabolism by only 4.5% within an hour of drinking. Brown concluded that this was too little to help with weight loss.
In addition, the scientist noted that Boschmann's work used direct calorimetry in a special chamber, and not an apparatus for indirect respiratory calorimetry, which is put on the head of the participant in the form of a dome or mask.
Perhaps Boschmann actually measured incorrectly, twice (in 2007 he again experimented in a respiratory chamber and got a 24% increase after 500 ml of cold water). But in 2011, another paper came out that tested calorie expenditure in obese children.
Here, as in Brown's experiment, an indirect calorimeter was used, but the results were similar to those obtained by Boschmann. Within 40 minutes of drinking 10 ml of cold water (4°C) per kilogram of body weight, the resting metabolic rate increased by 25%.
It is difficult to draw unambiguous conclusions, as the results vary and the topic requires further research.